The Arctic is warming three times as fast and the global average. This is mainly because melting of snow and ice exposes a darker surface and increases the amount of solar energy absorbed in these areas (albedo effect?). This significant regional warming leads to continued loss of sea ice, melting of glaciers and of the Greenland ice cap.
Status
Temperature
Various factors drive the earth’s climate to change continuously over many different time scales. Processes linked to continental drift have influenced atmospheric circulation, ocean currents, and the composition of the atmosphere over millions of years. A global cooling over the past 60 million years has changed conditions in the Arctic from an ice-free surface year round, to a surface completely covered with ice. Variations in solar radiation over many millennia as a result of alterations in earth’s orbit around the sun have created warm and cool cycles, leading to changes about half as large as those caused by continental drift. On a shorter time scale, the current interglacial period (Holocene) has seen brief periods of cooling caused by volcanic eruptions, weak variations in solar radiation, and probably other factors as well.
Climate in the Arctic
The Arctic has a cold climate. This is mainly because the earth’s axis is tilted relative to the sun and less solar energy reaches the polar regions. In addition, the Arctic is covered with snow and ice much of the year. Snow and ice have high reflectivity, albedo, which helps keep the Arctic cool.
The degree of warming seen in the Arctic since 1980 is twice that in the rest of the world. Temperatures in most parts of the Arctic have increased substantially in the past few decades, particularly in winter. The winter increase in Alaska and western Canada has been around 3–4°C over the past half century. Meteorological stations in the Norwegian High Arctic have also noted increasing temperatures. In Longyearbyen, the yearly average temperature increased by about 0.25°C per decade from when measurements started in 1912 through 2011, a slightly larger increase than for the Arctic as a whole over the same time period. Examination of available knowledge and evidence reveals that the years 2005–2011 have been the warmest ever recorded in the Arctic. In recent years, the warming trend has been strongest in fall and spring.
Research conducted by the Norwegian Polar Institute
Research at the Norwegian Polar Institute has advanced scientific knowledge about temperature in ancient times, for example through studies of microfossils in sea sediments and of ice cores.
By examining the tiny fossilised shells of foraminifers – organisms that are exquisitely sensitive to temperature – the Institute has contributed toward charting the relationship between species distribution and temperature; this information can then be used to interpret and calculate temperature trends back over time.
The Norwegian Polar Institute also studies ice cores from Svalbard and Antarctica to help chart temperature trends in ancient times. In a broader context, the data gathered through these projects contribute toward making climate models better.
The geographic distribution of warming in the Arctic strongly suggests that the decrease in sea ice coverage has enhanced the warming. The largest temperature increases have been recorded in the lower atmosphere over the marginal ice zone in autumn. This can be an indication that the Arctic has reached a threshold where solar radiation absorbed in the summer limits formation of ice during the following autumn and winter. Such positive feedback mechanisms enhance the increase in air temperature over the Arctic Ocean. Larger temperature increases have also been registered over the central Arctic Ocean after the ice cover has thinned and/or retreated earlier in summer. This contrasts with the situation earlier in the warming period, when warming over land was the dominating effect. The increased warming in the spring appears to be related to snow melting away earlier in recent years.
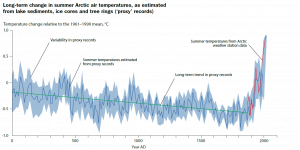
Long-term change in summer Arctic air temperatures, as estimated from lake sediments, ice cores and tree rings (“proxy records”). Figure: SWIPA
Comparison between temperature observations in the Arctic over a fifty-year period (1957–2006) and model predictions for the same period shows that the models are able to describe both the observed temperature increase in the Arctic and the degree of temperature rise. On average, the temperature in the Arctic as a whole has risen between 0.5 and 1.0°C in this period, according to both the models and the actual observations.Temperatures in the distant past (before thermometers) can be calculated by use of proxies? such as lake-bottom sediments, growth rings in trees, and ice cores. From this type of data we know that summer temperatures in the Arctic are higher today than at any time in the past 2000 years. The last time the polar regions were significantly warmer than they are now over an extended period of time was 125 000 years ago.
Temperatures in the Arctic are determined by a range of factors – both natural and man-made – that interact in complex ways and result in the changes we are currently seeing. Changes in sea ice and its influence on heat transfer between ocean and atmosphere, heat transport in the atmosphere, cloud cover and water vapour that influence long-wave radiation, soot on snow and higher concentrations of soot aerosols in the atmosphere: all these are fundamental conditions for how temperatures evolve.
Percipitation
Total precipitation in the Arctic in recent years has been about 5% higher than the average in the 1950s. This trend is not statistically significant, because year-to-year variability is large. However, both the amount of precipitation and the flow rate in rivers evoke the impression that the Arctic has been wet since 2000. The five wettest years since 1950 have all been during the 2000s. Increased precipitation has also been observed in Svalbard, and at Longyearbyen, the annual precipitation has increased by 2% per decade since measurements started there in 1912.
Precipitation is important in evaluating recent climate changes in the Arctic. There are indications of increased cloud formation in the Arctic, particularly low clouds in the summer; this coincides with a longer summer season and reduced sea ice. At present, our knowledge about clouds and cloud formation is still fraught with uncertainty.
Climate at sea
The capacity to absorb and store heat is much greater in the ocean than in land masses. This means that oceans have a cooling effect during periods of global warming. In times when the temperature falls, the opposite will occur: the oceans will release heat to the atmosphere and retard cooling. A large part of the global rise in temperature in recent decades is stored in the ocean.
The Arctic Ocean is warmed by inflow of warm water from the North Atlantic and the northern Pacific. On the Pacific side of the Arctic Ocean, this appears to be one of the causes underlying reduction in sea ice. From the North Atlantic, warm water comes to the Arctic in intermittent surges. One such surge of warm water flowed into the Arctic Ocean along the Siberian continental shelf in the mid-2000s. The next surge is expected to come through Fram Strait. The relationship between warm Atlantic water and melting sea ice on our side of the Arctic Ocean is more complex than on the Pacific side, and much research is being dedicated to this field.
Extreme weather events
In some places on the North American side of the Arctic, extreme temperatures and storms have been recorded. However, no consistent increase in storm activity in the Arctic as a whole has been recorded over the past half century.
Evaluating the observations
Today’s climate models are able to recreate with good accuracy the changes seen in the Arctic – both qualitatively and on a broader scale: global warming driven by increased emission of greenhouse gases. Nevertheless, some major changes come “unannounced” or long before they were expected, as in the case of the shrinking sea ice cover. The gradual effect of human influence, combined with major events in the atmosphere or ocean, or deviations caused by natural variability and modified by arctic feedback mechanisms: this is what leads to irreversible changes in the Arctic.
When observed changes in temperature are subdivided according to region and season, we see an increase of 1°C above and beyond natural climate variations (in each region and each season) throughout the Arctic; in some places and in some seasons, the increase is even larger.
The driving force behind the rise in temperature is the human contribution. No consistent patterns similar to this can be found during earlier warm periods.
Future scenarios
Details in the projected future climate in the Arctic vary between models, regions, seasons and expected future levels of greenhouse gas emissions – but there is nonetheless good agreement concerning the main features of the climate of the future.
There are three main sources of uncertainty in the global climate model projections of the future climate: large natural variability, uncertainty concerning future emission of greenhouse gases, and unresolved weaknesses in the climate models. Despite the uncertainty in the models, there is consensus in the research community, based on model projections, that the warming trend will continue to be stronger in the Arctic than in the rest of the world,
Temperature
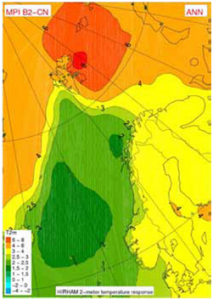
Expected change of yearly average temperature from the 30-year period 1961–1990 to the 30-year period 2071–2100, based on NorACIA’s regional climate model, NorACIA-RCM. Green designates the smallest expected temperature increase and red the largest expected increase. Note the sizeable rise in temperature expected in the easternmost parts of the Svalbard archipelago and the strong gradient across Svalbard. Photo: Førland et al. 2010
Model simulations show a general increase in average autumn and winter temperatures in the Arctic on the order of 3–6°C before 2080, and the models indicate that the warming over the Arctic in winter will exceed the global average temperature increase in the same period.
Projections made using results from the regional climate model NorACIA-RCM show that yearly average temperatures will increase about 1°C in coastal areas of the northern Norwegian counties of Nordland and Troms, and 1.5–2.0°C in the eastern parts of Finnmark and southwestern Spitsbergen by 2050. For the Svalbard archipelago, these calculations show a considerable difference in temperature increase from southwest (about 2°C) to northeast (over 4°C). Changes in sea ice coverage have a major impact on the geographic distribution of warming.
Simulations to the end of this century show that warming will continue after 2050, and for large parts of northern Norway, the temperature will have increased by 2.5–3.5°C before the end of the century, least in coastal areas in the west and most in Varanger and inner parts of Finnmark in the north. For Svalbard, the calculated increase in yearly average temperature is about 3°C in the southwest and about 8°C in the northeast. The model shows least warming in the summer and greatest warming in autumn and winter, particularly in inland areas. The ocean area between Svalbard and Novaya Zemlya is expected to experience a significant increase in air temperatures, particularly in the months from September to May. It is in these areas, where sea ice is expected to be replaced by open ocean, that the greatest increases in temperature will be seen. Farther south in the region, temperatures are expected to increase more over land than over sea.
Precipitation
Climate models generally show increased precipitation in the Arctic through to 2100, and predict a much larger percent increase in the Arctic than the global average. The increase is expected to be largest at high latitudes, and most conspicuous over northeastern Greenland, followed by the coast of Siberia and the Canadian archipelago. Seasonal variability is great, and the largest relative increase is expected in winter and autumn, with the smallest change in summer.
The predicted increase in precipitation in the Arctic is generally largest in the climate models that show most warming.
Only part of the additional precipitation will fall as snow; some will fall as rain owing to the expected warming and shorter spans of time with temperatures below freezing.
Calculations done with the regional climate model NorACIA-RCM show that large parts of northern Norway can expect annual precipitation to increase by 20–30% before the end of this century. In the northeastern parts of Spitsbergen the expected increase is as much as 40%. Precipitation will increase throughout the region in all seasons, but most in winter in spring. At present, little precipitation falls in winter on Spitsbergen, and the absolute increase is no more than a few millimetres. The simulations also show that there will be more days with relatively large amounts of precipitation throughout the region. Fewer days with heavy snowfall (over 10 cm per day) are expected in coastal northern Norway and the southwestern parts of Svalbard. Farther inland in northern Norway and in northern Svalbard the number of days with heavy snowfall is expected to increase.
Impacts
Ongoing and expected climate changes in polar regions over the next decades influence and will continue to influence atmospheric circulation, vegetation and the carbon cycle – and have impact on the climate system both within and outside the polar regions. The consequences of climate change in these areas are numerous. Sea ice melts, influencing the radiation balance in the global climate system through the albedo effect?. Warming may influence bottom water formation? through surface warming and increased input of fresh water; this would have impact on the “motor” in the ocean system, which in turn defines the framework for the world’s climate. Glaciers melt and contribute strongly toward rising sea levels. Thawing permafrost contributes to release of greenhouse gases (chiefly methane) that are presently “locked away” underground. Ecosystems are adapted to the climate in a certain area, and climate change will therefore influence ecosystems. However, the various ecosystems in the Arctic will react differently to such changes.
Climate change can influence transport and distribution of ecotoxins because the compounds’ reactivity, and their uptake and accumulation in organisms are all temperature-dependent.
Climate effects are simultaneous with other influences such as pollution, fishing, changes in land use, population increases, and changes in culture and economy. All these influences combined can amplify the impact on the health and well-being of both humans and ecosystems. Frequently the overall effect is greater than the sum of the individual factors, as we see for example in the case of pollutants, increased ultraviolet radiation and warmer climate. Which effects are most important and how they interact will depend on local factors in each individual region of the Arctic.
Also see the report: «Climate change in the Norwegian Arctic – Consequences for life in the north» (2011)
How do we measure climate change?
Several methods can be used to observe climate and climate change. Today’s scientists use sophisticated instruments and satellites to measure climate parameters. We can also look back through time by studying ice cores and sediment cores that provide indirect evidence about past temperatures and other climate conditions. Climate change is measured both globally and regionally.The most common climate observations are of temperature and precipitation. Other climate variables include humidity, wind speed and direction, air pressure, cloud cover and solar radiation.
In polar regions, observed changes in sea ice, snow cover and glaciers also tell us what is happening with the climate.
Ice sheets as climate archives
Glacier ice, particularly the ice in the inland ice sheets of Antarctica and Greenland, holds a treasure trove of information about climate in ancient times. The snow that once fell here contains information about ambient climate hundreds of millennia back in time. Tiny air bubbles trapped in the ice allow scientists to study how the composition of the atmosphere has changed with temperature over time.
One of the most important sources of information in these icy archives is cryptically called δ18O or dD. This is a measure of the relative concentration of different stable isotopes of oxygen in the water the ice crystals are made of. In simple terms, every time water evaporates from the ocean or falls as precipitation, the molecules of water (H2O) that contain certain stable isotopes are more likely to be involved. The exact fraction is temperature-dependent, so if we analyse the snow on the glaciers, we can create a time-line that tells us how temperatures in that area have varied. When this information is stored over long time spans, it becomes a climate archive.
As in all archives, accurate dating is important. Many different methods can be used to calculate the age of an ice core, and several are usually used in parallel. Horizons (layers) formed in conjunction with historic events are important in this context. Volcanic eruptions provide another important way of dating ice cores.
Climate components in the Arctic
The Arctic is characterised by distinct components consisting of water in frozen form: glaciers, snow, permafrost and iced-over water. These components together form the cryosphere. All parts of the cryosphere are sensitive to climate change, and they all play important roles in the global climate system.
Sea ice
Sea ice covers large parts of the Arctic Ocean in the winter. Some places are ice-covered year-round, but the edges of the ice cover melt in summer; ice floes break off and float away with the ocean currents. Overall, there is as much as three times more ice in winter than in summer.
The extent of the sea ice cover has decreased sharply over the past 30 years, and the ice is disappearing faster than the climate models have been able to project. The ice is also thinner; less of it is compact, massive multiyear ice. More specifically, the IPPC summary of available knowledge from 2013 shows that the extent of the sea ice has decreased by 3.5 to 4.1% per decade in the period from 1979 to 2012, and the amount of sea ice that has survived at least one summer season has decreased by 9.4 to 13.6% per decade over the same period.
It is quite certain that these changes in extent of sea ice are happening faster than before, and the fastest changes are seen in the summer and autumn months. The records suggest that the thickness of ice in the Arctic Ocean has decreased by an average of 1.3 to 2.3 metres between 1980 and 2008. In some parts of the Arctic this means that ice-free conditions last up to two months longer. These pan-Arctic trends are apparent also in waters near Norway, the Barents Sea and Fram Strait.
The series of observations reported to MOSJ (Environmental Monitoring of Svalbard and Jan Mayen) show distinctly negative trends in ice coverage throughout the monitoring period. Moreover, although these observations show great variability in the thickness of ice passing through Fram Strait, both the variability and the thickness have decreased in recent years.
Taken overall, the sea ice in the Arctic is expected to continue to have less coverage and be thinner at all times of the year, albeit with regional variations. An ice-free Arctic Ocean in summer may become reality before the middle of this century.
In the global climate system, the area with ice cover will decrease with time owing to altered radiation balance (because of the albedo effect). This will in turn have impact on other processes and driving forces that set the framework for the global climate.
Changes in sea ice will have impact on the habitat of ice-associated species such as plankton that have evolved to live at the edge of, in, or under the ice, as well as fish, seabirds, seals, whales and polar bears.
Glaciers
The Fifth IPCC Report (2013) summarising available knowledge shows that almost all of the world’s glaciers are shrinking. In the Arctic, Alaska and northern Canada are among the areas where glaciers have lost most ice mass over the past decade. Several parts of the Greenland ice cap have lost mass in the past two decades, and loss of mass is spreading to new parts of this vast ice sheet. The rate of loss is increasing. Surface melting and glacier calving each contribute about half of the loss, and both melting and calving are on the increase. The glaciers are expected to continue to lose mass – somewhere between 15 and 85% before the end of the century, depending on the future RCPs.
Melting glaciers contribute strongly to rising sea level. The average sea level rose about 0.19 m between 1901 and 2010. Glaciers and ice sheets have contributed to this rise. Average sea level is expected to continue to rise, and models predict an increase of 0.53-0.97 metres by 2100. So far, most of the increase has been caused by thermal expansion of seawater: the warmer the ocean, the greater its volume. Melting of glacier ice is the second largest contributor to sea level rise so far, but it can become the main contributor in the future. Changes in mass balance of the Greenland ice sheet are expected to continue contributing toward a rising sea level.
Ice on rivers and lakes
Of the thousands of lakes and rivers in the Arctic, most are covered with ice at least six months per year. Although there are regional differences in the Arctic, the period when these waters are ice-bound is shorter now than it was 100 years ago. Ice forms later in the autumn, and breaks up earlier in the spring.
Snow
Snow
In the Arctic, the ground is covered with snow three quarters of the year. Both satellite observations and observations on the ground show that the snow cover in the northern hemisphere has decreased over time, particularly in the spring. These changes are most obvious in the places that are warmest, but that are nevertheless normally snow-covered for parts of the year. The snow cover in the northern hemisphere will continue to shrink through the rest of this century, by as much as 25% depending on the future RCP?s.
Less snow means a longer growing season, and may lead to changes in vegetation, for example more shrubs and less lichen. That means less food for some animals and more food for others. In this way, alterations in snow season have impact on the entire ecosystem. Higher temperatures in the Arctic will also lead to more precipitation falling as rain in the winter months. When rain falls on snow, ice forms on the surface, preventing grazers such as reindeer and musk oxen from reaching forage under the snow. Read more about the effects of climate change on polar ecosystems
The snow cover, like sea ice, is an important factor in maintaining radiation balance in the global climate system through the albedo effect?). Read more about processes and drivers
Permafrost
Much of the land in the Arctic is permafrost, ground that is permanently frozen; in some places permafrost can also be found under the seabed. Observations and measurements show that the temperature in the permafrost has risen in most places since the 1980s, albeit with large regional differences. In some places the permafrost is now 2°C warmer than it was 20-30 years ago. In parts of Scandinavia and Russia, the active layer of earth that lies atop the permafrost and that thaws in the summer, has become as much as 20 cm thicker, and there have been major reductions in permafrost in the European parts of Russia. The total area of the northern hemisphere with surface permafrost is expected to decrease by as much as 80% by the end of this century, depending on the future RCP?.
Thawing permafrost releases greenhouse gases (mainly methane) that are currently “locked” in the ground. When permafrost thaws it will also affect the hydrological cycle in the area. Small lakes and tarns will usually dry out when the permafrost thaws and their water drains away.